madman
Super Moderator
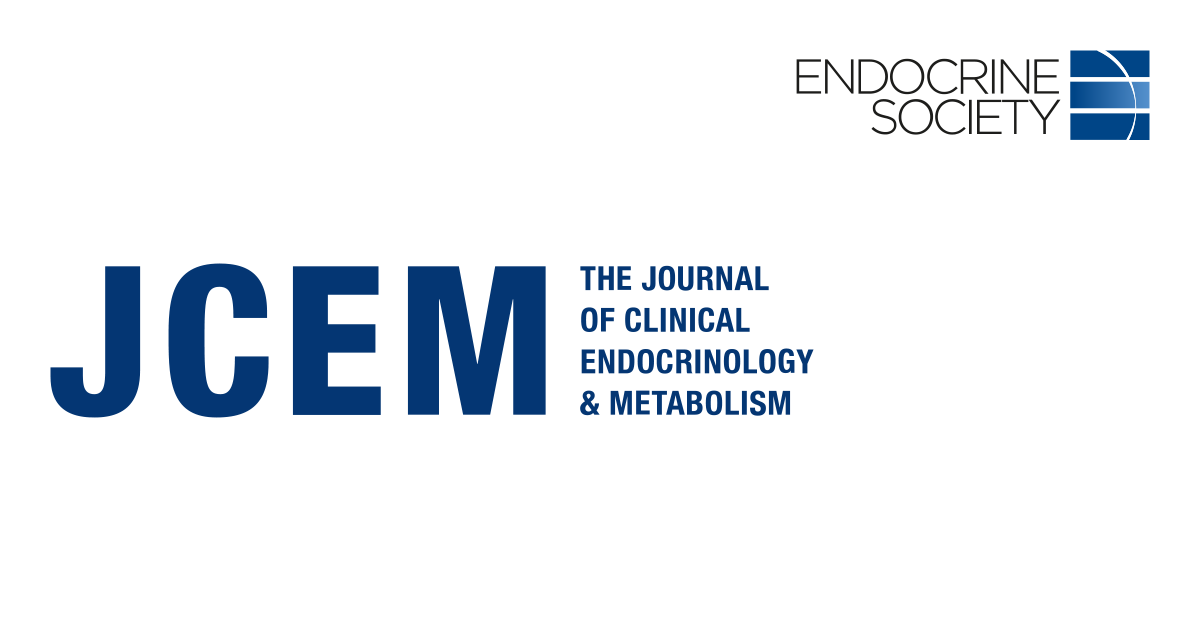
Testosterone and Erythrocyte Lifespan
AbstractContext. Endogenous and exogenous androgens increase circulating erythrocytes and hemoglobin but their effects on erythrocyte lifespan is not known

Conclusions
We conclude that hypogonadal men have reduced erythrocyte lifespan and acute androgen-induced increase in circulating erythrocyte lifespan may contribute to the well-known erythropoietic effects of androgens, but longer-term effects require further investigation to determine how much they contribute to androgen-induced increases in circulating hemoglobin
Introduction
It has long been recognized that endogenous androgens such as testosterone and its exogenous synthetic androgen analogs stimulate circulating erythrocyte numbers and increase blood hemoglobin concentration (1). As a result, blood hemoglobin is 10-12% higher in healthy men than women (2), a difference originating at male puberty due to the 20-30-fold increase in testosterone production in males compared to females among whom circulating testosterone concentrations remain unchanged (3, 4). Similarly, circulating erythrocyte and hemoglobin concentrations are increased by exogenous androgen treatment (5-7) including therapeutic doses (7-13) or illicit androgen abuse (14); however, the mechanism is not fully understood. The mechanism is believed to involve androgen stimulation of renal erythropoietin (Epo) leading to increased synthesis of early-stage erythrocytes (reticulocytes) and thereby increased blood hemoglobin; however, other molecular mechanisms involved, including those regulating the body’s iron economy (15), are not fully understood. For example, testosterone increases iron availability for erythropoiesis (16) but can worsen anemia in iron-deficient mice (17). Circulating erythrocytes numbers and blood hemoglobin concentration represent an equilibrium between production (erythropoiesis) and erythrocyte turnover or degradation so can be affected by changes in marrow erythropoiesis or extramedullary degradation of erythrocytes. For example, mistaken attribution of Epo resistance (18) may occur when reduced RBC survival is compensated by increased marrow erythropoiesis with or without changes in Epo secretion or sensitivity.
One mechanism is that testosterone stimulates renal Epo secretion which then stimulates bone marrow erythroid production via the increased circulating Epo. This mechanism is supported by some (19, 20), but not all (21, 22), studies showing testosterone increases Epo concentrations. Other studies suggest a decline in Epo concentrations with time (23) and minimal or no increases after prolonged testosterone treatment for 20 weeks (21) or 36 months (22). These discrepancies may be reconciled by experimental studies showing testosterone treatment increased renal Epo production and circulating Epo concentrations and reticulocytes within days of Epo treatment (24). Over time, however, circulating Epo concentrations establish a new equilibrium with circulating testosterone (1). Aromatization of testosterone to estradiol to activate estrogen receptors is not required (8, 9, 25), and may partially antagonize (24), the erythropoietic effects of testosterone.
Regulation of iron-containing hemoglobin is strongly connected with the body’s iron economy. Testosterone administration suppresses hepatic production of hepcidin, an iron sensing regulatory protein that reduces iron absorption when body stores are adequate. In that role it serves as an essential protective mechanism for the body which has no excretory mechanism for excess iron, which exerts toxic oxidative effects when tissue stores are excessive (26). Hepcidin binds to intestinal epithelial ferroportin blocking gut iron uptake when the body’s iron stores are sufficient. Conversely when hepcidin levels fall ferroportin is free to facilitate intestinal iron uptake (15). Hepcidin is subject to negative feedback by erythroferrone, secreted from erythroblasts (27) and which suppresses hepcidin (24). However, neither hepcidin nor other hypoxia-sensing mechanisms are required for the androgen-mediated increase in erythropoiesis (16, 28) leaving aspects of androgen regulation of circulating hemoglobin still not fully explained.
In addition to direct androgen stimulation of erythropoiesis, testosterone may increase blood hemoglobin via other mechanisms such as prolonging erythrocyte lifespan. However, no direct studies of erythrocyte lifespan in testosterone deficiency (hypogonadism) or following testosterone administration are available. Hence, we investigated erythrocyte and reticulocyte life span in humans and mice to determine whether testosterone influences erythrocyte13 lifespan. In humans, we employed a novel, validated, non-invasive breath test to measure erythrocyte lifespan. The breath test measures alveolar carbon monoxide (CO) concentration which, together with blood hemoglobin concentration, provides a simple but accurate estimate of erythrocyte lifespan (29-31). Using this breath test method, we studied hypogonadal or transgender men before and for 18 weeks after commencing standard dose T treatment. In complementary studies of mice, we investigated circulating half-time of reticulocytes and mature erythrocytes using in vivo biotin labelling of erythrocyte cell surface biomarkers together with flow cytometric modelling to monitor the disappearance over time of characteristic epitopes of immature (reticulocytes) and mature erythrocytes. For a comprehensive view of acute and chronic androgen effects on murine erythrocyte lifespan, we also measured circulating reticulocyte and erythrocyte half-lives in intact or orchiectomized male mice with and without testosterone treatment, intact females with and without testosterone treatment as well as AR knockout (ARKO) male and female mice.
Discussion
The present study investigated testosterone effects on erythrocyte and reticulocyte lifespan in humans and mice aiming to add a new dimension to mechanistic studies of the well-known physiological and pharmacological effects of androgens to increase circulating erythrocytes and blood hemoglobin concentration. Previous studies indicate that erythropoietic effect of androgens is at least partly due to increasing renal Epo secretion whereby higher circulating Epo and/or sensitivity enhanced erythrocyte production (1). The converse mechanism of androgen mediated changes in erythrocyte turnover and lifespan have not been studied systematically in generally healthy individuals. Here we provide human and mouse evidence that acute, but possibly not chronic, testosterone administration increases erythrocyte lifespan. The possible mechanisms for such testosterone effects are speculative but may be due to the androgenic effect of increasing erythropoiesis causing release from the bone marrow reserves of longer lasting young erythrocytes which then contribute to longer overall survival times compared with the older circulating erythrocytes. This may also involve changes in erythrocyte membrane composition due to testosterone treatment (39)
Older literature on androgen effects on human erythrocyte lifespan are from the pre erythropoietin era (pre-1990) when androgens were used to treat anemia due to chronic renal failure. That anemia has complex mechanisms including erythropoietin deficiency and/or resistance, hemolysis with reduced erythrocyte lifespan and uremic toxicity suppressing erythropoiesis (40). In the pre-erythropoietin era, a single study measured erythrocyte survival (by 51-chromium erythrocyte labelling) reporting that testosterone treatment did not increase the reduced erythrocyte lifespan in uremic men on dialysis (41). Another study randomized 72 dialysed uremic men to treatment for up to 6 months with nandrolone 100mg weekly (n=4) or untreated controls (n=3) (42). The expected androgen-induced erythropoietic changes were observed however erythrocyte survival was not measured; nevertheless, for two patients increased erythrocyte survival was inferred based on discrepancies between increased erythrocyte mass without increases in erythron iron turnover, although other interpretations were not excluded. Ultimately, studies of androgen effects on the reduced erythrocyte survival in uremic men on dialysis is not comparable with the present study in generally healthy individuals.
In our human studies, the most prominent finding was that, compared with non-transgender, non-hypogonadal healthy controls, hypogonadal and transgender men had reduced erythrocyte lifespan even prior to testosterone treatment. This difference was mediated via increased alveolar CO concentrations and reduced hemoglobin concentrations without between-group differences in reticulocyte count and unrelated to age or birth sex. The finding that erythrocyte lifespan was increased at 6 weeks after start of testosterone treatment but returned to baseline by 18 weeks whereas blood hemoglobin and serum testosterone remained elevated at 6 and 18 weeks, indicates that the transient increase in erythrocyte lifespan by the Levitt formula could not be solely due to the increased hemoglobin. The lack of difference in testosterone effects on reticulocytes at 6 and 18 weeks may be at least partly due to the short lifespan (~1 day) of reticulocytes. Erythrocyte lifespan did not differ between male and female controls. However, erythrocyte lifespan in hypogonadal men was significantly lower than in eugonadal male controls. Surprisingly, transgender (F2M) men before treatment had lower erythrocyte lifespan than female controls, which was unexpected based on their common female birth sex. Whether non-prescribed hormone or other treatments among F2M explains this difference is unknown.3 Although erythrocyte lifespan is reduced in numerous conditions such as iron deficiency (43),diabetes (44, 45), chronic renal failure with or without hemodialysis (18, 44, 46), rheumatoid arthritis (47), anemia of chronic illness (47), prosthetic heart valves (48) and certain drugs (ribavirin, interferon) (30, 49), the influence of male hypogonadism or of testosterone treatment have not been reported previously.
Iron deficiency could limit the blood hemoglobin response to testosterone through a shortened erythrocyte lifespan but also by limiting erythrocyte and hemoglobin synthesis via effects on hepcidin (16, 28). Testosterone administration reduces circulating hepcidin (19) thereby increasing gut iron absorption and enhancing hemoglobin synthesis, a mechanism inhibited by iron deficiency (50, 51). As none of our participants were iron deficient and together with the strong testosterone-induced hemoglobin response, insufficient iron availability was not a factor in this study.
The mouse studies used biotin labelling of circulating erythrocyte surface proteins (52) to estimate immature (reticulocytes) and mature erythrocyte half-life in the circulation (53). The water-soluble, non-cleavable biotin ester labels accessible outward facing lysine residues on the surface proteins of the erythrocyte forming a covalent amide bond between the biotin’s carbonyl and epsilon amino group of lysine residues in membrane proteins (52). Using specific streptavidin-labelled anti-mouse antibodies with suitable flow cytometry gating we could simultaneously estimate the disappearance rate (half-life) of multiple biomarkers on the mouse erythrocyte and reticulocyte cell surface. These gave consistent estimates that murine reticulocyte half-life was not influenced by sex, genetics (ARKO vs wild-type) or androgen exposure except for a larger effect of T than DHT treatment in wild-type mice and reduced lifespan for female but not male ARKO mice, indicating an effect on erythrocyte lifespan not mediated by the androgen receptor. The ineffectiveness of DHT is consistent with a 1980 study of androgen-resistant and wild-type mice in which in vivo and in vitro T treatment increased murine erythroid colony formation but DHT did not (54). For erythrocyte markers, both T and DHT treatment were associated with longer erythrocyte half-lives for both markers (except in wild-type female for the CD41- marker), whereas neither sex nor orchidectomy in wild-type mice had significant effects. The similar effects of T and DHT on both erythrocyte markers effectively exclude any influence of aromatization of T to estradiol to act on estrogen receptors in explaining the androgen mediated effects on erythrocytes. The present findings have shorter erythrocyte half-life estimates than those of Dholakia et al (55) with the difference likely due to our use of specific erythrocyte epitopes rather than unselective total erythrocyte numbers in flow cytometry. Our present half-life setimates were consistently shorter using the more specific Ter119+ erythrocyte marker than the CD41- marker which identifies all non-leukocytic (CD41+) cells.
The original prompt for this investigation was the discrepancy in the T4DM study between testosterone-induced improvement in acute glycemia but unchanged HbA1c, a conventional measure of chronic hyperglycemia (56). HbA1c is an integrated measure of chronic glycemia over the lifespan of erythrocytes due to the cumulative effects of non-enzymatic glycation of hemoglobin by prevailing blood glucose concentrations. Hence HbA1c is increased by sustained higher ambient blood glucose concentration as well as by longer erythrocyte lifespan whereas shorter erythrocyte lifespan and lower glycemia decreases HbA1c (57, 58). One possible explanation for the discrepancy in the T4DM study was that testosterone administration might increase erythrocyte lifespan obscuring any decrease in chronic glycemia. The present study did not observe a significant increase in HbA1c over the first 3 months of testosterone treatment nor were increased reticulocyte numbers observed (which could reduce average erythrocyte lifespan) providing little support for the possibility that testosterone may increase HbA1c at least by mechanisms involving the AR or short-lived reticulocytes. Longer term effects of testosterone treatment on HbA1c warrant further investigation to determine the degree to which changes in erythrocyte lifespan contribute to the well-known androgen-induced increases in blood hemoglobin. Further studies are underway to determine whether chronic testosterone treatment can rectify the shortened erythrocyte lifespan observed in this study prior to testosterone treatment in hypogonadal men.
Strengths of this study is the utilization of novel, minimally invasive testing without use of radioactive tracers to estimate erythrocyte lifespan. Limitations of this study include its small sample size although using efficient statistical methods significant findings were obtained. Although there were similarities the human and mouse data were not completely consistent and further studies are required to further investigate the discrepancies. For example, while androgen effects of T or DHT are evident in some mouse and human groups, the discrepancy between male and female ARKO mice is puzzling as indicating an effect on erythrocyte lifespan not mediated via the androgen receptor, which otherwise mediate most all androgen effects.